At the heart of BeCoM stands the enhancement of the physical representation of ice cloud and the treatment of ISSRs, which improves the prediction of persistent contrails, hence allowing the integration of contrail schemes in the existing policy framework to enable eco-efficient trajectories.
The successful delivery of BeCoM’s aim and objectives will be managed via seven work packages (WPs), including five technical WPs, one on exploitation and dissemination (WP6), and one on management (WP7) (see section 3). WPs 1 to 5 are organized to address the objectives of BeCoM (see section 1.1.1). The five scientific WPs are:
WP1: Operational & new measurements & characterization
WP2: Cloud Physics & weather models & assimilation
WP3: Evaluation of data and model predictions
WP4: Climate optimized trajectories
WP5: Policy-driven Flight Planning
Routine measurements of humidity at cruise level
Despite our statement that it is currently impossible to predict the formation of persistent contrails at the right place and time, past airborne measurement campaigns have demonstrated good experiences in forecasting situations prone to contrail generation and persistence. The explanation of this apparent contradiction lies in the scales. For a measurement campaign, one needs predictions for larger regions and time periods (e.g., “there will be enough ice super-saturation west of Ireland on Wednesday afternoon”). However, for contrail avoidance (in particular strongly warming ones), one needs the prediction in more spatial and temporal detail (e.g., “Flight levels 320 to 340 in sector NN are closed between 1600 and 1700 UTC”).
Relative humidity (RH) is a highly variable field, with variability caused by large- and small-scale dynamics and cloud processes. The RH variability appears on smaller scales than temperature (i.e., the autocorrelation length of RH is much shorter than that for temperature), which makes forecasting RH and supersaturation much more challenging than forecasting temperature. Therefore, we need more humidity data for cruise levels.
To tackle this issue, the lack of humidity data at cruise altitudes for data assimilation needs to be remedied, ideally by much more in-situ measurements from commercial aircraft than are currently available. In complement, ground-based Lidar is a unique opportunity to measure with a vertical resolution of 100 meters the water vapor with cirrus occurrence either from the meteorological process or induced by aircraft contrails. Such observations derived with the scale of 5-10 minutes integration will enable us to calculate the degree of supersaturation. These observations will also be compared with space observations to quantify the differences induced by the lack of resolution.
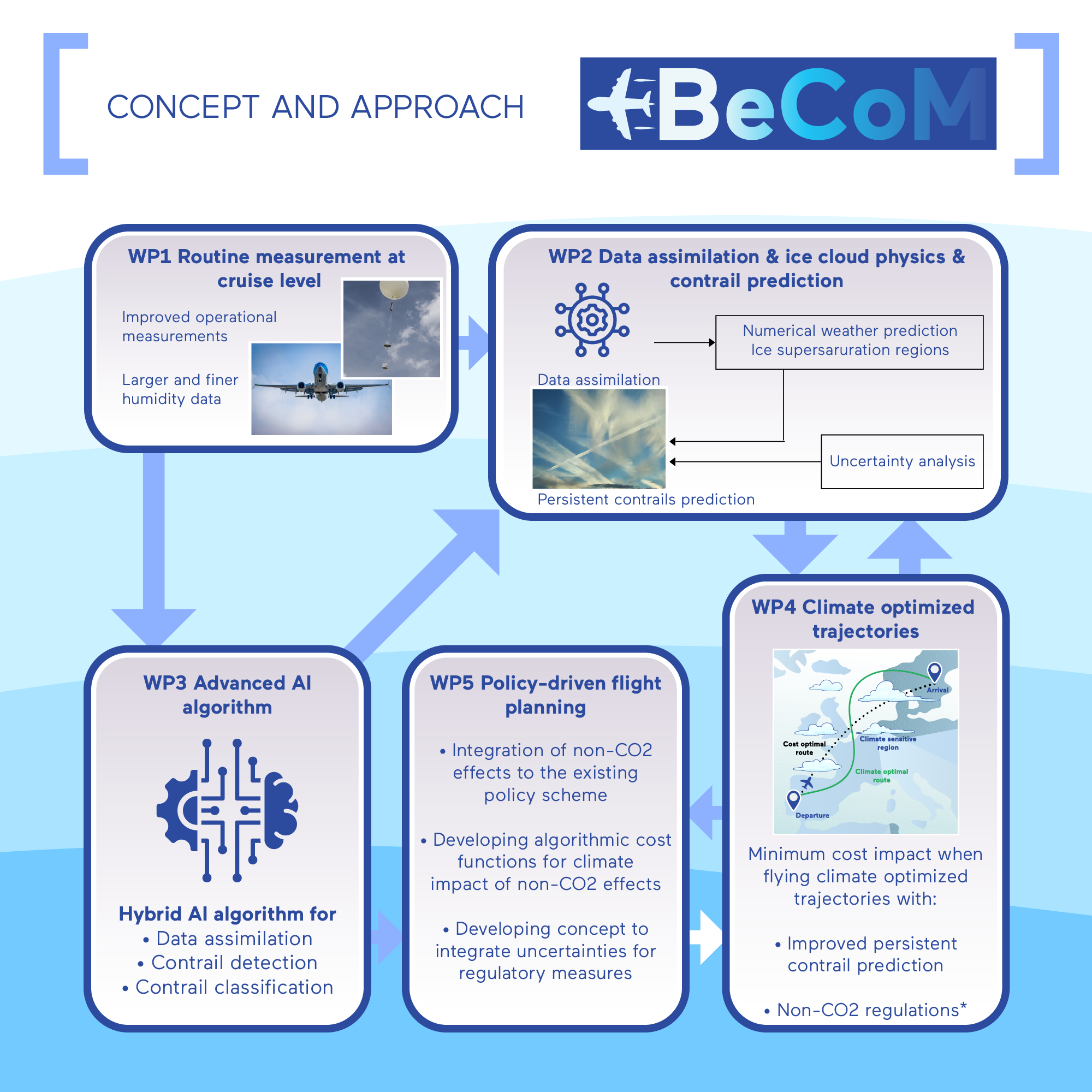
The treatment of ice supersaturated regions in NWP
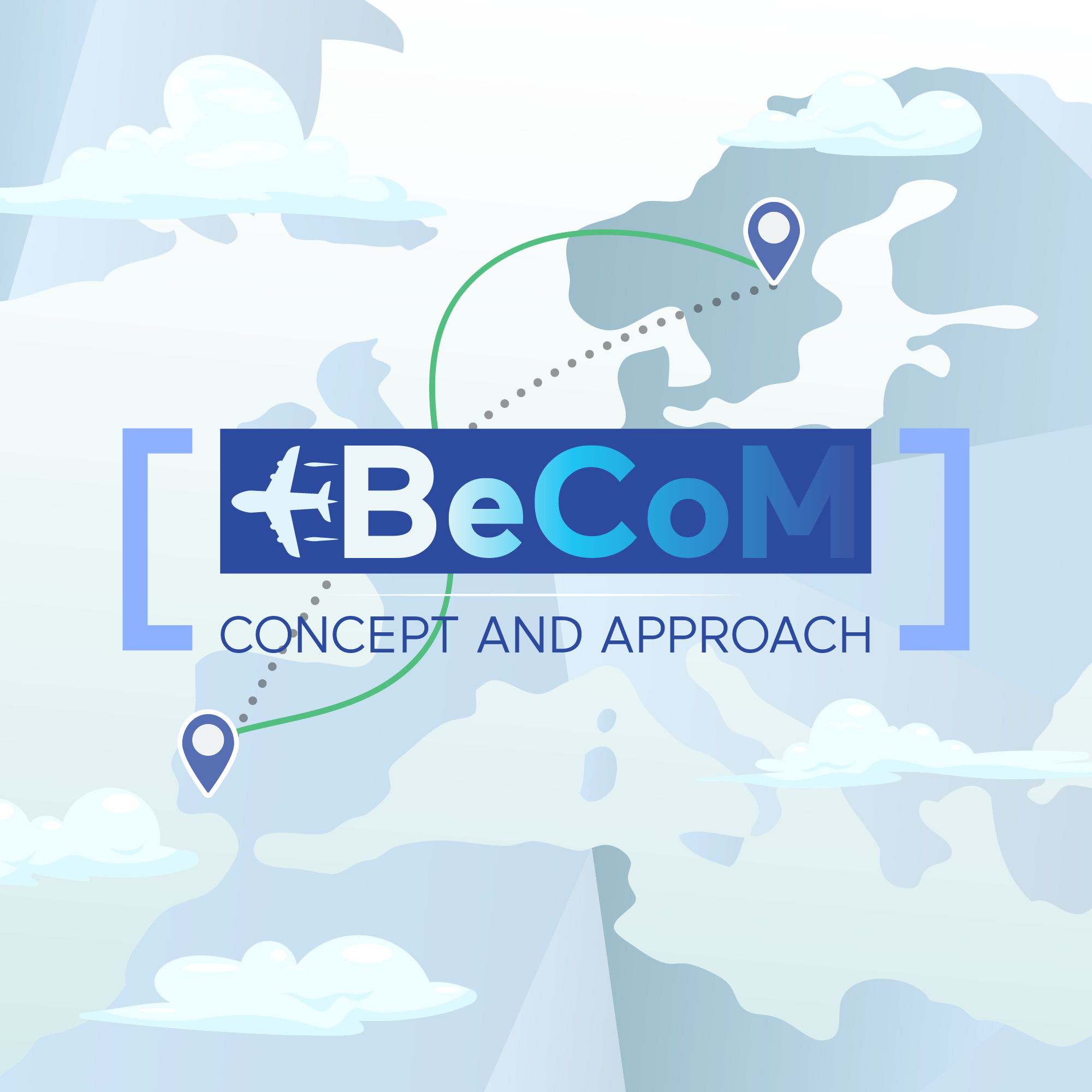
The formation of persistent contrails needs ice super-saturation, although they can survive for a while in sub-saturated air. While the contrail formation can be predicted with some success, the prediction of persistent contrails is problematic, which is mainly associated with the poor prognosis of RH fields and, in particular, the ice super-saturation regions in NWP and climate simulations. Specifically, the current weather forecast models cannot capture the ice super-saturation at the correct location and time, resulting in underestimating the frequency and degree of ice super-saturation.
Previous research (Gierens, 202135) shows that the prediction skills for contrail persistence increase significantly with a slight decrease of the threshold of relative humidity concerning ice (RHi) from >100% to >99%. Such unsteady prediction behaviors are strongly related to the inadequacies in the numerical weather forecast model concerning the treatment of ice cloud physics and its humidity background. Therefore, the weather forecast centers need to improve the representation of ice cloud (cirrus and contrails) physics to tackle the problems of predicting persistent contrails.
Current ice cloud modules of NWP models generally employ certain simplifications for the formulation of the ice cloud physics, making it computationally not too expensive. The simplifications lead to the low bias of RHi values from NWP models. The latter problem is aggravated when some ice cloud modules assume that cirrus formation starts at ice saturation, which is an unrealistic assumption that should be dropped. We can exploit the progress in computing power to switch unrealistic simplifications to a more realistic ice cloud physics that would also lead to improved treatment of ice supersaturation in cloud-free areas around clouds.
Here we will design a new concept that does not require the in-cloud supersaturation to be consumed entirely within a one-time step. Alternatively, the growth rate of the ice mass will be proportional to its thermodynamic force, i.e., the degree of supersaturation. Accordingly, a cloud will not vanish entirely as soon as there is subsaturation.
Ice cloud formation will not occur immediately at ice saturation but at a temperature-dependent threshold supersaturation which might further depend on the nature of the aerosol present. (Such refinements are not essential for the concept). A current example of this is the integrated forecast system of the ECMWF.
This concept can be refined later, for instance, to incorporate the ice crystal number concentration within the clouds. However, the main developments for this task are independent of such refinements. The concept will be formulated in a box-model version, but it will be tested in a 3D environment.
Scientifically based contrail mitigation via policy-driven trajectory optimization
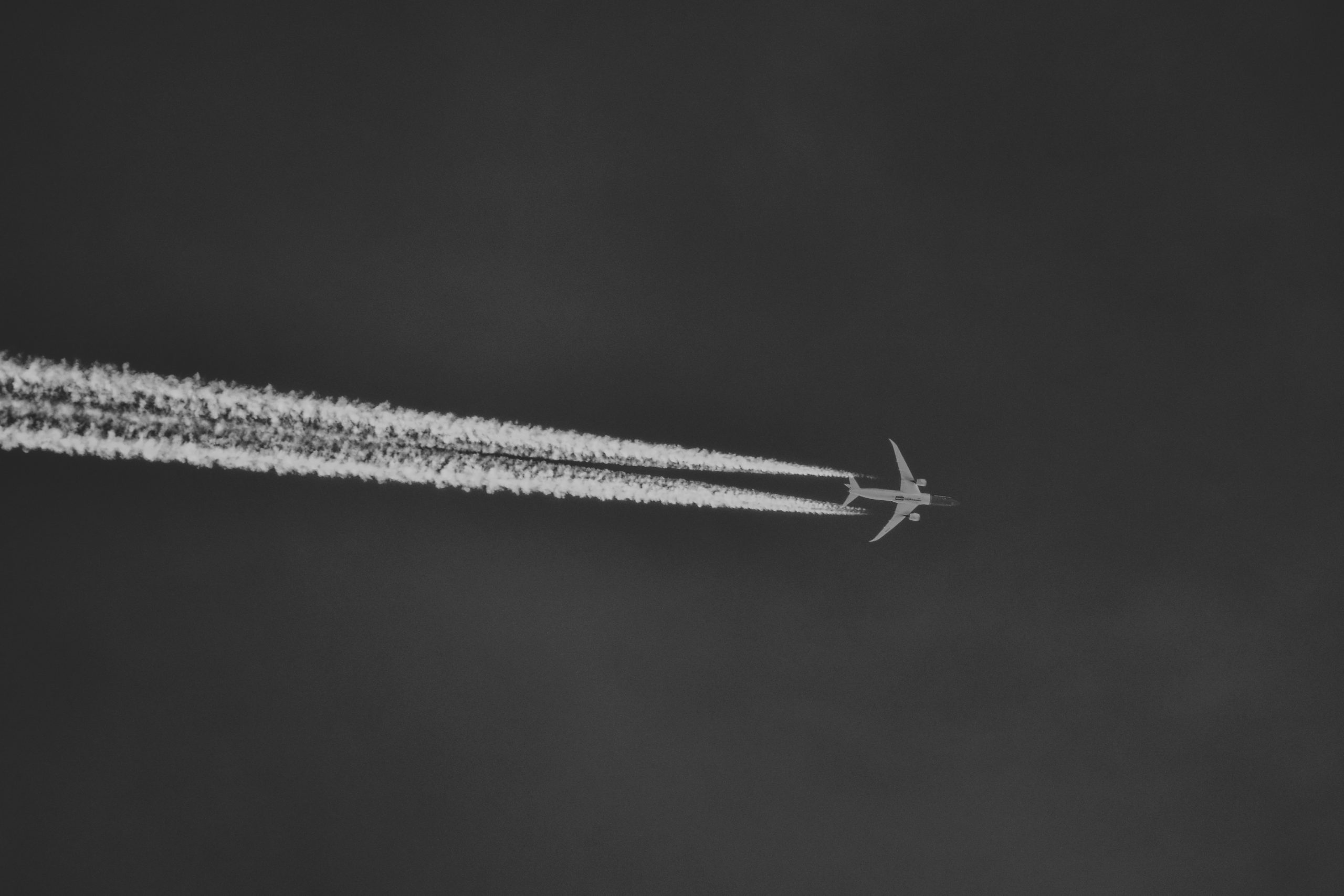
Grewe et al. (2017)31 and Teoh et al. (2020)36 demonstrate the possibility of contrail avoidance with minimal cost penalties. The latter showed that about 80% of contrail radiative forcing is caused by only 2% of flight distances for a set of Japanese flight data. They demonstrated that about 20% of contrail energy forcing could be avoided by rerouting without increases in fuel consumption and corresponding CO2 emissions. Nevertheless, given the inaccurate prediction of persistent contrails, the mitigation gains from contrail avoidance, and therefore, the reduction of aviation’s climate impact as a whole, require revaluation.
In this context, the improved persistent contrail skills in BeCoM will be implemented in the Earth-System model EMAC/Contrail (see model description in Table 1.6), which is further coupled with the air traffic simulator (EMAC/AirTraf) to optimize flight trajectory over a long period of, e.g., one year, concerning the contrail avoidance, the consequent climate impact, and the cost. We focus on identifying the changes in contrail avoidance resulted from the use of the improved contrail prediction skills developed in WP2, therefore, evaluating the impact of the contrail predictions skills on the reduction potentials of aviation’s climate impact. The challenge is correctly implementing the new contrail prediction approach into the existing contrail model, which will be resolved via close communications and collaborations between WP2 and WP4. In addition, we apply the non-CO2-based measures from WP5 to the EMAC/AirTraf (Table 1.6) to perform a similar procedure as the above. Accordingly, we evaluate the changes in climate impact reductions between a free-run (i.e., no regulatory measures) and regulated trajectory optimization.
Further development of policy and regulations, including non-CO2 effects
As previous studies have indicated, non-CO2 effects can be effectively mitigated by adjusting the daily aircraft routing. However, as these mitigation efforts are linked with additional expenses, it is essential to integrate non-CO2 effects into existing policies and regulations. For implementing climate-cost-efficient routings, a detailed review of non-CO2 policy instruments will be conducted first. Different concepts, e.g., CO2 equivalent (CO2e) approaches that incorporate non-CO2 effects into existing policy instruments (e.g., EU, CORSIA, and flanking policy instruments) for non-CO2 effects, climate-restricted flight altitudes, or climate-charged airspaces will be reviewed thoroughly. This review is a basis to deduct specifications for integrating price-based non-CO2 policies into flight planning and trajectory optimization. For accounting for non-CO2 effects, an algorithmic concept characterizing weather-dependent climate charges of aircraft emissions at a given location and time (algorithmic 4-D cost functions for climate change) is developed. This includes the elaboration of (1) a monetarization logic that quantifies the amount of emission certificates to be surrendered or respectively the level of emission charge/tax to be paid per climate impact of CO2, H2O, NOx, and contrail-cirrus, as well as (2) a workflow for accounting existing non-CO2 uncertainties. To achive robust climate-sensitive flight planning, parametric analysis of algorithmic cost functions for climate change is conducted.
BeCoM’s measurements focus and available technologies
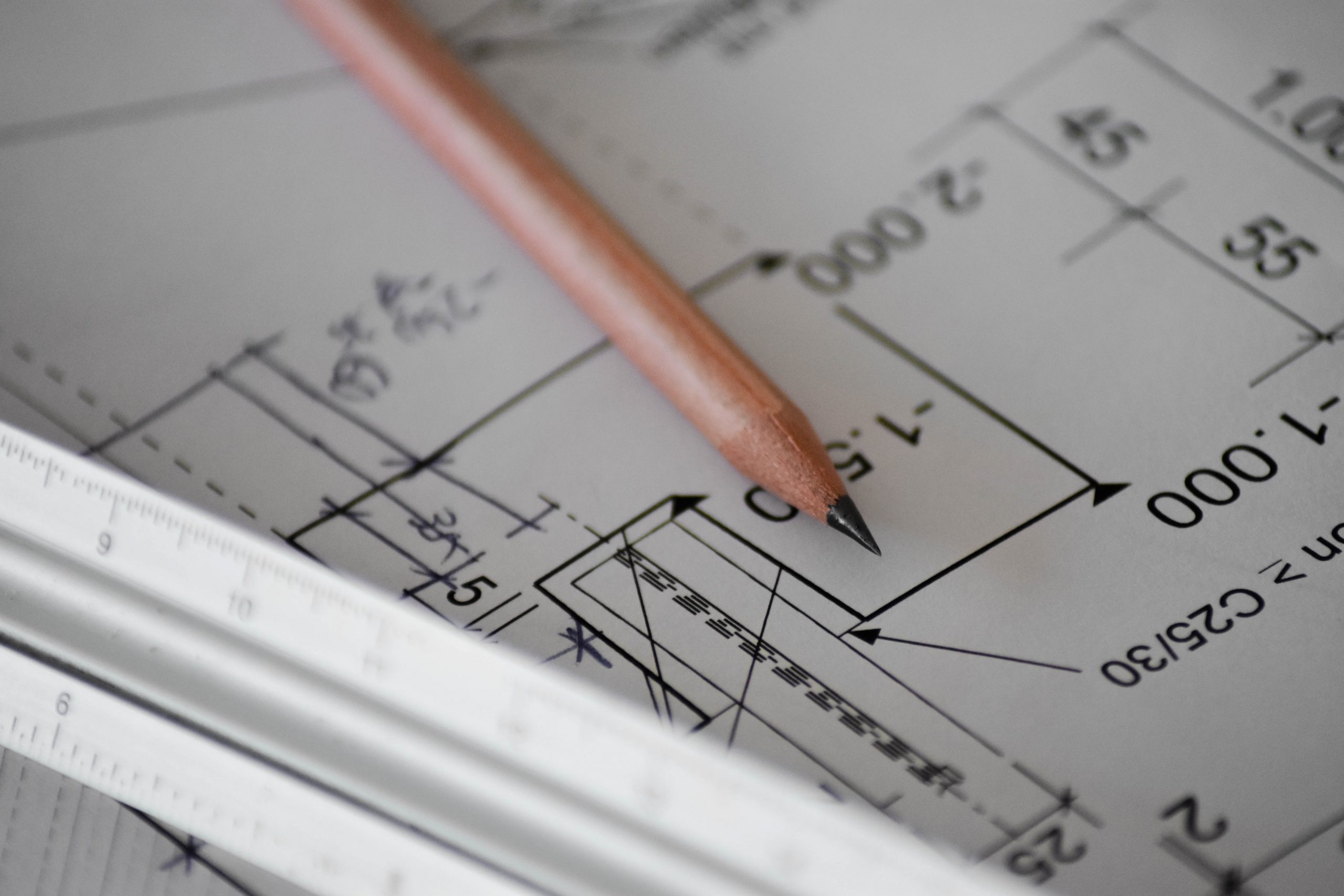
Measurements Domain Consortium member, expertise
Short description
Lidar Research network CNRS
Radiosondes Meteorology CNRS
Fash sondes Research upper atmosphere CNRS
AMDAR Operational on commercial aircraft DLR
BeCoM’s models, and the underlying assumptions :
Tool name Domain Consortium member, expertise
Short description
EMAC Climate impact / Meteorology DLR/TUD, development and validation
EMAC is an open access and open source state-of-the-art community Earth-System model based on the European Centre for Medium Range Weather Forecasts – Hamburg (ECHAM) climate model as part of the Modular Earth Submodel System (MESSy) Atmospheric Chemistry model. Sub-models describe tropospheric and middle atmosphere processes and their interaction with oceans, land, and human influences. An extensive model description and validation is given by Jöckel et al. (2016) and in an open-access special issue.
EMAC submodels AirTraf and Contrail Combined Aviation and climate Impact DLR/TUD, development and validation; Trajectory optimization
Within the EMAC model (see above), AirTraf is a submodel coupled at every time step to the EMAC. It enables global air traffic simulations on long-time scales, considering the effects of local weather conditions on aviation emissions and contrails (Yamashita et al., 202039). Air traffic information comprises Eurocontrol’s Base of Aircraft Data (BADA, Revision 3.9) and the International Civil Aviation Organization (ICAO) engine emission databank. Fuel use and emissions (H2O and NOx) are calculated by the total energy model based on the BADA methodology and DLR fuel flow method. AirTraf uses the persistent contrail coverage (predicted from the EMAC/CONTRAIL submodel (Supplement to Grewe et al. 201440)) as inputs. The flight trajectory optimization is performed by a Genetic Algorithm with respect to different objectives (e.g., cost and contrail avoidance). EMAC/AirTraf will be used in WP 4 for analyzing the effectiveness of persistent contrail prediction skills and the avoidance of persistent contrails subject to the regulations.
Data available to the beCoM consortium :
Domain Name of the database (origin/user)
Aircraft performance Base of Aircraft Data (EUROCONTROL)
Engine fuel & emissions ICAO Engine Exhaust Emission Databank (EASA)
Meteorological data ERA-5 meteorological data (ECMWF)
High Resolution EPS (ECMWF)
Model forecast, radar data, current measurements, and observations (DWD)
Air traffic and flight movements DDR2 Demand data repository (EUROCONTROL)
Contrail detection &assimilation Satellite observation (DLR), ground-based images (DWD), Lidar data (CNRS)